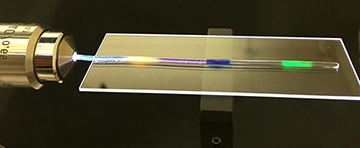
By injecting dyes into their stretchy, biocompatible hydrogel optical fiber, the Harvard–MIT team was able to construct a strain sensor that could quantify where, and how much, the fiber had been stretched based on changes in optical loss at differing wavelengths through the fiber. [Image: Courtesy of the researchers]
Optogenetics has opened up new windows to the brain’s neuronal activity, through the ability to stimulate photosensitive proteins with fiber-delivered light. The activity, however, has largely been restricted to research studies in laboratory animals. Those same studies have in some cases raised the tantalizing possibility of actual therapies involving light delivered to the brain, over the long haul—but the physical characteristics of optical fiber make it a poor match for the soft biological tissue of the brain in a permanent implant.
A team of scientists from the Massachusetts Institute of Technology (MIT) and Harvard University, USA, and Tsinghua University, China, has now developed a flexible, highly stretchable and biocompatible approach to making optical fiber that could help resolve that dilemma (Adv. Mater., doi: 10.1002/adma.201603160). To do so, they turned to a material with a long pedigree in tissue-engineering, drug-delivery, and wound-dressing applications: hydrogels.
Biocompatible but brittle
Hydrogels are polymerized networks of absorbent, aqueous gels that are highly flexible and have some similarities to natural tissue. They’ve been investigated in the past for photonic applications such as light delivery to tissues, blood monitoring, and even optogenetic therapy. But one stumbling block has been the fact that common, synthetic hydrogels tend to be fragile and brittle, making them a poor fit for long-term applications, such as implants or wearable technology, that might have to deal with some stress and strain over time. Drawing the gels into optical fiber with a core–clad structure that enables total internal reflection constitutes a still greater challenge.
To step up to that challenge, the Harvard bio-optics lab of OSA Member Seok-Hyun Yun teamed up with researchers under MIT mechanical engineering professor Xuanhe Zhao. Yun’s group had worked for some years toward fabricating optical fibers from hydrogels, and were able to devise fibers that could achieve light transmission—but that couldn’t handle much strain. The researchers saw some promise in the mechanically tougher hydrogels being developed across town by Zhao’s operation, which had developed chemical techniques to bond hydrogels into various combinations and even into stretchable electronics.
Clever chemistry
The Yun–Zhao team focused in particular on an alginate-polyacrylamide hydrogel under development at Zhao’s lab, which seemed to have the right combination of toughness and optical properties to be a good optical-fiber candidate. The team found that it could tweak the refractive index of the hydrogel by adjusting the concentration of acrylamide in the polymer mix.
The researchers settled on a concentration of 40 percent acrylamide for the fiber core and 20 percent for the cladding. To make the fiber, they squirted a precursor solution of the core recipe into a silicon tube mold, and polymerized the material by treating it with UV light. Then they extracted the fiber from the mold and dipped it into the cladding solution to provide the outside layer. Some clever chemistry using conjugation chemicals—“molecular-level glue,” in the words of one of the paper’s authors, Hyunwoo Yuk of the MIT group—enabled the team to cross-link the core and cladding layers into a strong and flexible combination.
A bio-strain gauge?
By shining a 532-nm laser into the end of the fiber, the team established that the flexible hydrogel fiber did indeed guide light, and were able to quantify its optical properties and loss characteristics. They also found that the hydrogel fibers could be straightforwardly attached to conventional, silica-based multimode fibers through an intermediate silica connector. And the new hydrogel fiber was tough; a millimeter-diameter fiber, for example, could stretch up to 730 percent before rupturing.
The researchers used that combination of optical transfer and elasticity to create a simple fiber strain sensor from the material. They treated different parts of the fiber with different colored dyes, and sent light through. As the fiber is stretched, the elongation increases the amount of light absorbed by a given color, which can be measured by the loss at specific wavelengths at the other end of the fiber. “This could be an implantable or wearable strain gauge,” Yuk noted—perhaps with applications such as monitoring progress and range of motion as an injured limb heals.
Looking out further, the researchers believe that the new hydrogel fiber could find use in implanted long-term wearable and implantable sensors and diagnostics, to keep an eye on cancers and chronic diseases. The team thinks that the material could even deliver long-term optogenetic stimulation and treatment to targeted regions of the brain, and in other areas where “high flexibility, stretchability, and biocompatibility are required.”